Research
The development of medical technology is essential for people to live healthy and secure lives, and medical polymer materials underpin advanced medical technologies such as nanomedicine, immunotherapy, and regenerative medicine. Kojima Laboratory designs and fabricates medical polymer materials that function “strongly,” “gently,” and “beautifully.
- Strong → medical polymer materials with high therapeutic effect
- Gentle → patient-friendly (fewer side effects) medical polymer materials
- Beautiful → Smartly functional, material-designable medical polymer materials
Aimed Research
Currently, biomaterials research is dominated by methods to improve existing materials or to fabricate better materials through exhaustive studies. In other words, there is currently no clear design guideline for creating biomaterials that express the desired function. The Kojima Laboratory aims to create materials that can contribute to actual medical treatment as well as to establish design guidelines for functional biomaterials.
Research on polymeric materials for medical applications is interdisciplinary research. Kojima has research backgrounds in polymer materials science and molecular biology. In addition, he will conduct research in collaboration with laboratories in various fields such as chemistry, physics, biology, and medicine.
At Kojima Laboratory, our key phrase is “like makes like. If you like what you do, you can enjoy working on even seemingly difficult tasks. Why don’t you join us in searching for something interesting and something you can get absorbed in?
Dendrimer
Polymers are commonly used materials for medical applications. Biopolymers such as proteins can be controlled in structure and sequence, but the molecular weight, composition, sequence, and structure cannot be clearly defined for synthetic polymeric materials. Kojima Laboratory uses dendritic polymers called dendrimers. Since dendrimers are synthesized by organic synthesis rather than polymerization, their molecular weight, end groups, and structure can be clearly defined. In addition, various compounds can be introduced by binding to the numerous reactive functional groups on the surface or by encapsulating them in the internal space, making it possible to fabricate multifunctional materials (Figure 1). Furthermore, due to the high density of terminal groups on the surface, an aggregation effect of bound molecules can be expected. By taking advantage of these characteristics of dendrimers, we aim to fabricate functional biomaterials and obtain material design guidelines.
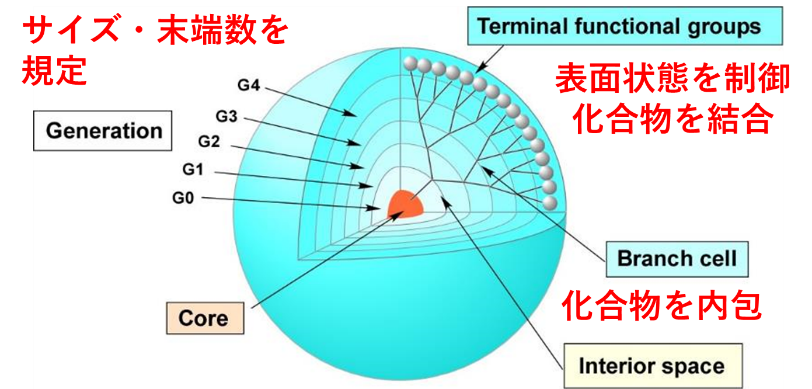
Specific Research
Strong medical polymers
Delivery to lymph nodes and immune cells (T cells) – Contribution to immunotherapy
Immunity is a system that protects the body from external enemies. In recent years, immunotherapy, which treats diseases by activating immune cells and teaching them information about the disease, has been attracting attention. Since immunotherapy uses its own biological system, it is also a treatment with smaller (gentler) side effects than chemotherapy, which uses artificial drugs.
Lymph nodes are the site of immune response because of the large number of immune cells present in them. Since lymph nodes are small tissues and immune cells are accumulated inside them, it is said that large particles cannot reach the immune cells inside. Therefore, dendrimers with a particle size of a few nm are useful for delivery to immune cells in lymph nodes. In our previous studies, we have found that modification of anionic molecules at the terminus allows them to migrate into lymph nodes (Fig. 2). We have also shown that the uptake behavior into immune cells differs depending on the terminal structure. With previous techniques, delivery to the interior of T cells, which play an important role in immune responses, has been difficult, but carboxy-terminal dendrimers bound with phenylalanine (Phe), a hydrophobic amino acid, were found to be taken up by T cells in lymph nodes (Figure 3). We are investigating the correlation between the structure of these dendrimers and their uptake behavior by T cells, as well as drug and nucleic acid delivery to T cells using these dendrimers. Dendrimers that migrate to lymph nodes and are not taken up by immune cells are also being investigated for use in drug delivery imaging to cancer metastasized to lymph nodes.
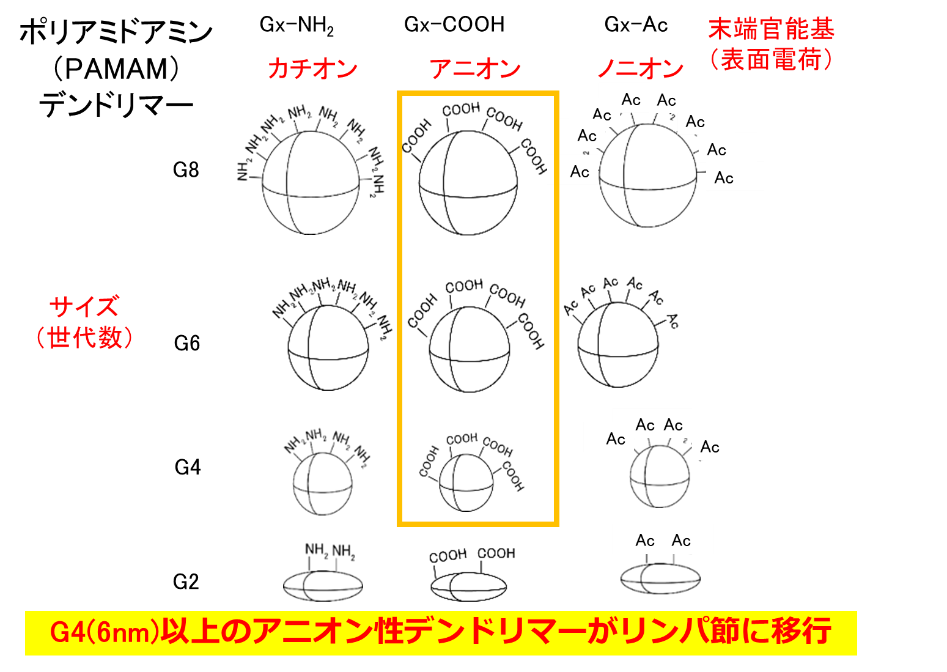
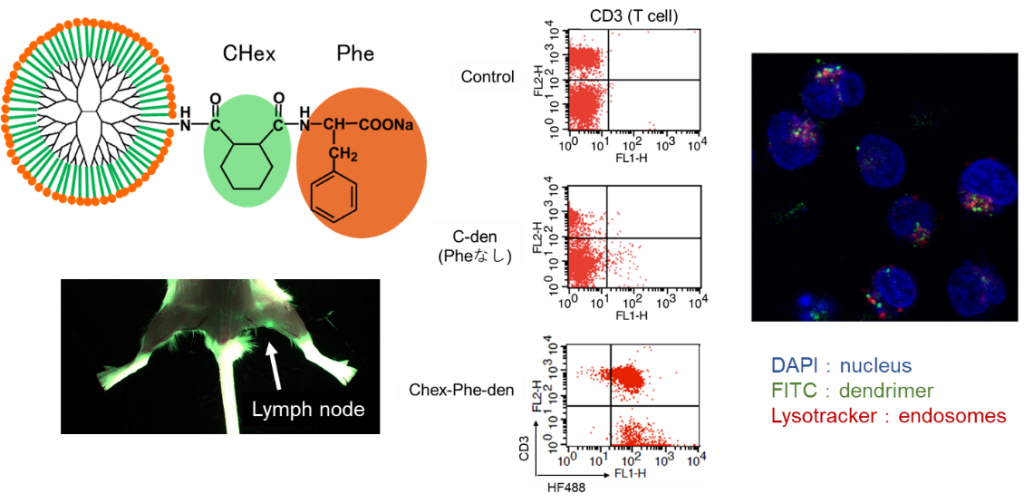
Representative Research Results
- Y. Niki, C. Kojima, et al, Nanomedicine, 11, 2119-2127 (2015).
- H. Shiba, C. Kojima, et al, J. Mater. Chem. B, 10, 2463-2470 (2022).
Selected as the cover image of Volume 10, Issue 14, published on April 14, 2022. - Y. Nishimoto, C. Kojima, et al, Polymers, 12, 1474 (2020).
- C. Kojima, et al, Macromol. Biosci., 23, 2300139 (2023).
Selected as the cover image of Volume 23, Issue 141, published in November, 2023. - K. Nagai, C. Kojima, et al, Bioorg. Med. Chem. Lett., 33, 127726 (2021).
Gentle medical polymers
Correlation between Tumor Accumulation and Hydration State of Biocompatible Nanoparticles – Correlation between Physical Properties and Delivery Function
To reduce the side effects of cancer chemotherapy, nanoparticles need to accumulate at the tumor site. Because of the larger vessel wall gaps in neovascular vessels found in tumor sites compared to normal sites, biocompatible nanoparticles with blood retention properties are known to passively accumulate in tumors without active interaction with the tumor (this is known as the EPR effect). Biocompatible nanoparticles are prepared by modifying the surface of nanoparticles with biocompatible polymers (such as polyethylene glycol (PEG) and zwitterionic polymers), and their blood retention varies depending on the modification rate and chain length. In our previous studies, we have prepared PEG-modified dendrimers and shown that they can carry various drugs (Fig. 4) and exhibit blood retention. The hydration states of PEG-modified dendrimers with different number of bonds have been investigated by thermal analysis (DSC method) and spectroscopy (FT-IR method). In polymeric materials for medical devices, hydration state is said to correlate with biocompatibility (intermediate water theory), and in PEG-modified dendrimers, which are DDS nanoparticles, the hydration state also correlates with tumor accumulation (Figure 5). PEG-modified dendrimers with different chain lengths, number of bonds, and number of generations have been prepared, and the correlation between hydration state and biocompatibility is being verified.
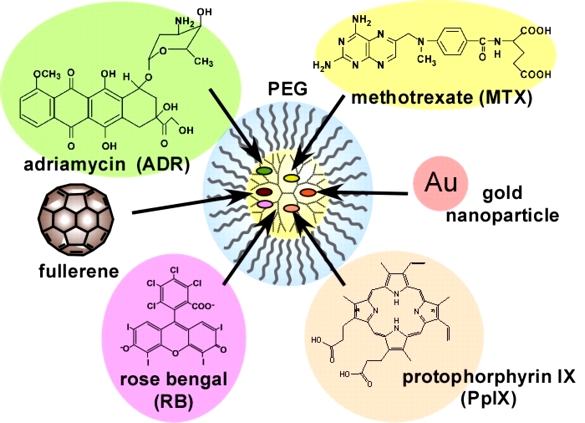
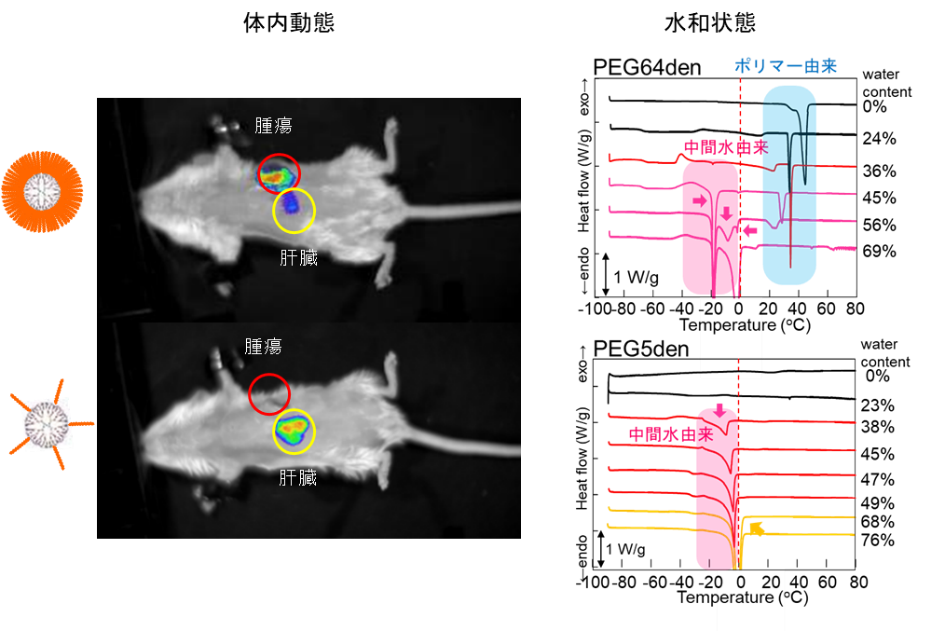
Representative Research Results
- C. Kojima, et al, Bioconjugate Chem., 11, 910-917 (2000).
selected for the 25th anniversary collection. Cited more than 500 times. - C. Kojima, et al, Int. J. Pharm., 383, 293-296 (2010).
- A. Tsujimoto, C. Kojima, et al, Mater. Sci. Eng. C, 126, 112159 (2021).
Beautiful medical polymers
Fabrication of Stimuli-Responsive Materials Responsive to pH, Temperature, Light, and Enzymes -Explorations in Nanomedicine and Regenerative Medicine
By introducing ionic or hydrophobic moieties at the ends of dendrimers, materials that respond to pH and temperature can be fabricated. Peptides and proteins that can be cleaved by enzymes can be used to create materials that are responsive to enzymes. In addition, gold nanoparticles can be loaded with photoresponsive properties (surface plasmon resonance, X-ray scattering ability, and photothermal properties that vary with particle size and aggregation state). This photoresponsiveness can be deployed in imaging and photothermal therapy, so materials containing gold nanoparticles can be used for both therapeutic and diagnostic (theranostics) applications. Furthermore, by combining various stimuli-responsive properties, functional materials such as stimuli-responsive DDS, DDS in response to cancer metastasis, light-irradiated cell separation materials, and optical sensors have been fabricated (Figure 6).
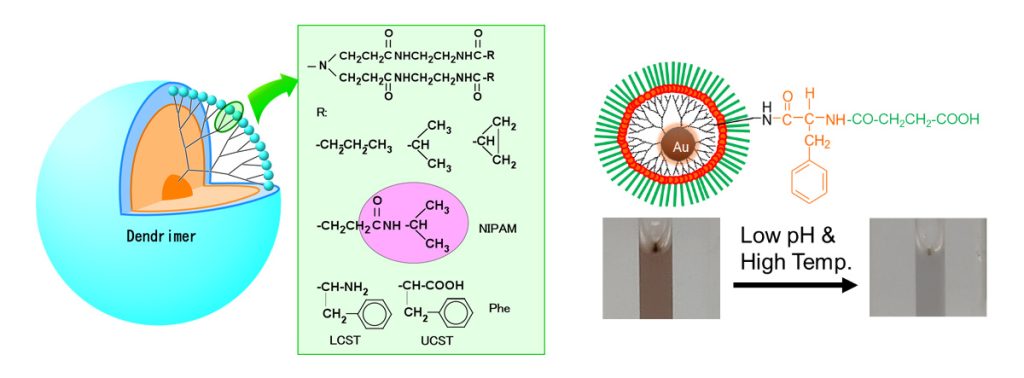
Representative Research Results
- Y. Haba, C. Kojima, Angew. Chem. Int. Ed., 46, 234-237 (2007).
- M. Tamaki, C. Kojima, RSC Advances, 10, 10452-10460 (2020).
- C. Kojima, et al, ChemNanoMat, 8, e202100442 (2022).
Selected as the cover image of Volume 8, Issue 3, published in March, 2022. - C. Kojima, et al, Macromol. Biosci., 17, 1600341 (2017).
- C. Kojima, et al, Acta Biomaterialia, 9, 5673-5680 (2013).
- Y. Umeda, C. Kojima, et al, Bioconjugate Chem., 21, 1559-1564 (2010).
- C. Kojima, et al, Nanotechnology, 21, 245104 (2010).
Highlighted in “nanotechweb”
Preparation of Artificial Proteins – Non-Denatured Proteins
Proteins are useful as functional materials, but they are difficult to synthesize chemically and are denatured and deactivated by pH, temperature, and solvents. We have created artificial proteins by bonding peptides and amino acids, which are substructures of proteins, to the ends of dendrimers of the same size. We have produced collagen and elastin pseudo-molecules (Fig. 7) and demonstrated that they function as protein-mimetic materials that do not denature, and we are also developing DDS and other applications that take advantage of the support function of the dendrimer core moiety.
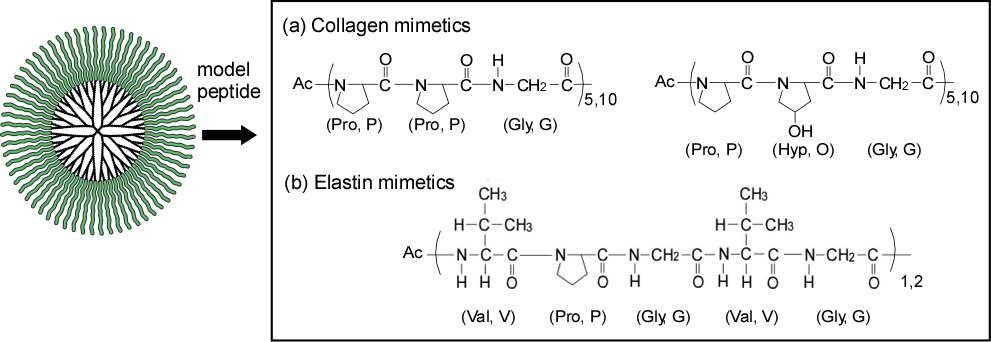
Representative Research Results
- C. Kojima, et al, J. Am. Chem. Soc., 131, 6052-6053 (2009).
- C. Kojima, et al, Soft Matter, 7, 8991-8997 (2011).
- C. Kojima, et al, Biopolymers:Peptide Sci., 100, 714-721 (2013).
- D. Fukushima, C. Kojima, et al, Colloid Surf. B-Biointerfaces, 132, 155-160 (2015).
Biological Tissue Transparency -3D Tissue Imaging
Tissues are generally sectioned and observed in two dimensions, but this method loses three-dimensional information when the tissue is sectioned, making it impossible to obtain a complete image of the tissue. Therefore, attempts are being made to make tissues transparent and observe them in their original three-dimensional form. There are various methods for tissue transparency, and we have conducted research to improve the polymer gel used in the CLARITY method. Ionic polymer gels, especially those with a zwitterionic structure, have been used to achieve tissue transparency in a shorter time. We believe that this technique may be used for cancer treatment and diagnosis.
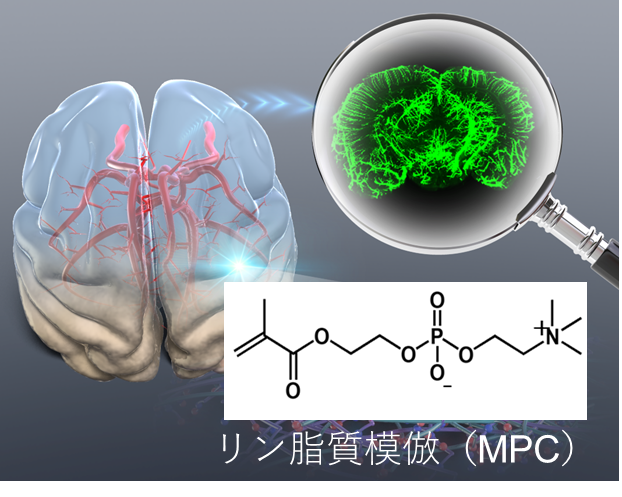
Representative Research Results
- C. Kojima, et al, Macromol. Biosci., 21, 2100170 (2021).
Selected as the cover image of Volume 21, Issue 9, published on September 18, 2021. - Y. Ono, C. Kojima, et al, J. Biomed. Mater. Res. B, 107, 2297-2304 (2019).